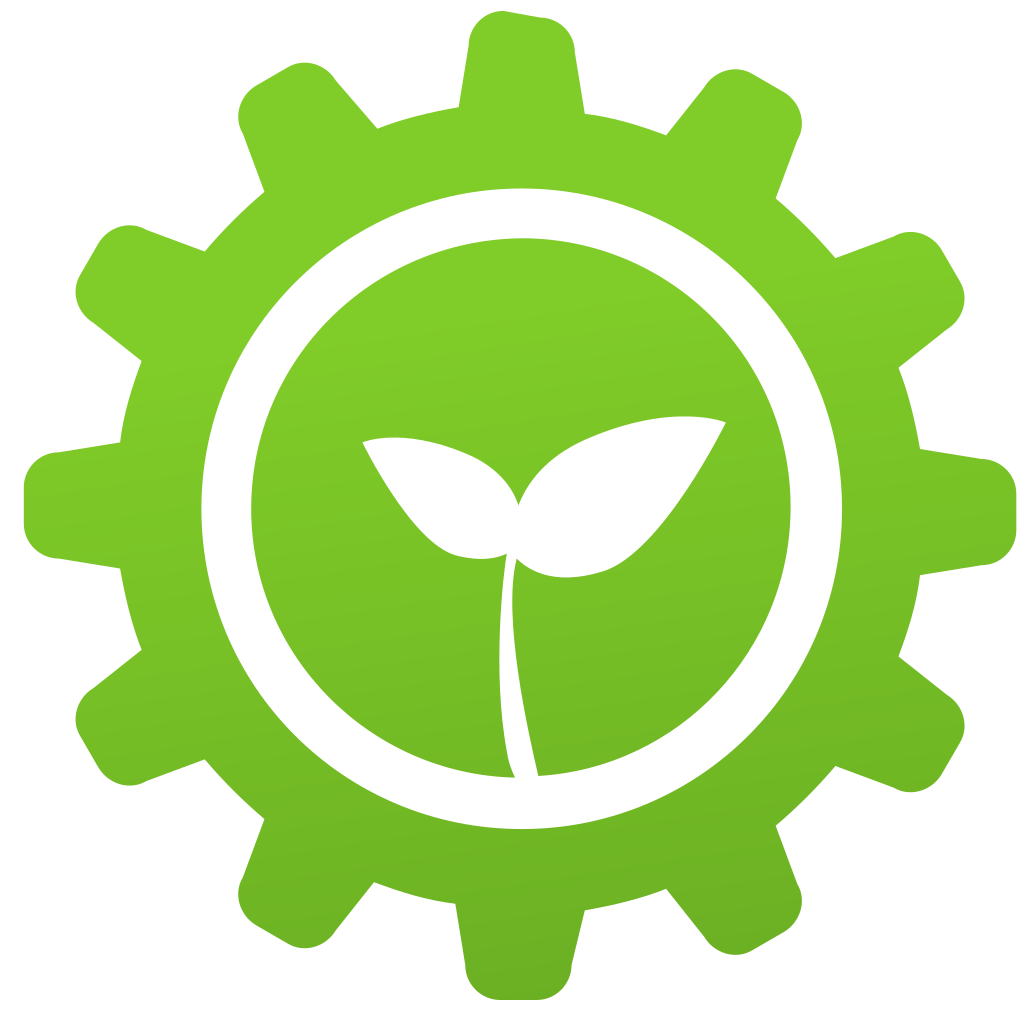
Methane Catalytic Cracking: A Promising Path to Clean Hydrogen Production
Resumo
The paper discusses methane catalytic cracking (MCC) as a potential method for hydrogen production that emits no CO2, thus offering a cleaner alternative to steam methane reforming. MCC involves decomposing methane (CH4) into hydrogen (H2) and solid carbon using a catalyst like nickel, iron, or copper. This process is promising because the resulting solid carbon can be used or stored, rather than released as CO2. Catalyst deactivation due to carbon deposition is a challenge, yet researchers are exploring regeneration techniques like steam and air regeneration. Fluidized bed reactors are identified as a suitable technology for MCC due to their advantageous heat and mass transfer and continuous operation capability, although particle attrition and design complexity are noted challenges. Kinetic and modeling studies have not yet converged on a standard model but continue to evolve. The paper suggests that while MCC is not currently economically competitive, its prospects may improve with advancements in catalyst and reactor technologies, especially if carbon capture becomes mandatory for other methods and if the solid carbon byproduct can be valorized. The development of MCC technology could significantly contribute to reducing the carbon footprint of hydrogen production if research continues to overcome existing technical and economic hurdles.
Artigo aberto completo
Methane Catalytic Cracking: A Promising Path to Clean Hydrogen Production
In the quest for cleaner energy solutions, hydrogen has emerged as a frontrunner in the race to decarbonize our energy systems. However, the current dominant method of hydrogen production - steam methane reforming - still results in significant carbon dioxide emissions. Enter methane catalytic cracking, a process that promises to produce hydrogen without the unwanted CO2 byproduct. This innovative approach is gaining traction in research circles and could potentially revolutionize the hydrogen production landscape.
The Basics of Methane Catalytic Cracking
Methane catalytic cracking is a process that breaks down methane (CH4) into hydrogen (H2) and solid carbon. The reaction takes place in the presence of a catalyst, typically a metal such as nickel, iron, or copper, supported on materials like alumina or silica. The process can be summarized by the following reaction:
CH4 → C + 2H2
This seemingly simple reaction holds immense potential. Unlike steam methane reforming, which produces CO2 as a byproduct, methane cracking yields only hydrogen gas and solid carbon. This carbon can potentially be used in various applications or safely sequestered, making the process virtually carbon-neutral.
Catalysts: The Heart of the Process
The choice of catalyst plays a crucial role in the efficiency and economics of methane cracking. Nickel has emerged as the catalyst of choice due to its high activity and relatively low cost. However, researchers are continually exploring other options to improve performance and longevity.
One interesting development in this area is the EU STORMING project, which is developing innovative structured reactors heated using renewable electricity to convert both fossil and renewable methane into CO2-free hydrogen and valuable carbon nanomaterials for battery applications. This project exemplifies the potential synergies between methane cracking and other emerging technologies.
The Catalyst Conundrum: Deactivation and Regeneration
While the initial activity of catalysts like nickel is promising, they face a significant challenge: deactivation. As the reaction progresses, carbon deposits on the catalyst surface, forming filaments that can eventually encapsulate the active sites and reduce efficiency. This deactivation process is one of the main hurdles in the commercialization of methane cracking.
To address this issue, researchers have been exploring various regeneration techniques. Steam regeneration has shown particular promise, with studies demonstrating that it can fully restore catalyst activity. Air regeneration is another option, although it comes with the risk of local hot spots that can damage the catalyst.
The Physics of Carbon Formation
Understanding the formation of carbon filaments is crucial to optimizing the methane cracking process. Current theories suggest that carbon atoms diffuse through the metal catalyst particle, driven by temperature or concentration gradients. These atoms then deposit at the metal-support interface, forming filamentous structures.
This process is both a blessing and a curse. While the carbon filaments can be valuable products in their own right, their growth also leads to catalyst deactivation. Balancing these competing effects is a key challenge in reactor design and operation.
Reactor Technology: Fluidized Beds Lead the Way
When it comes to reactor design for methane cracking, fluidized bed reactors have emerged as a promising option. These reactors offer several advantages, including excellent heat and mass transfer characteristics and the ability to continuously add or remove catalyst particles.
In a typical setup, catalyst particles are suspended in a rising stream of methane gas. As the reaction progresses, spent catalyst can be continuously removed and regenerated, while fresh catalyst is added to maintain activity. This continuous operation is crucial for industrial-scale hydrogen production.
However, fluidized bed reactors also present challenges. The constant motion of particles can lead to attrition, breaking down the catalyst over time. Additionally, ensuring uniform fluidization and avoiding channeling or slugging in the bed requires careful design and operation.
Kinetics and Modeling: Unraveling the Complexity
To optimize methane cracking reactors, researchers need a deep understanding of the reaction kinetics. Over the years, various kinetic models have been proposed, ranging from detailed mechanistic descriptions to simplified global rate equations.
These models typically account for the initial methane decomposition steps, carbon diffusion through the catalyst, and filament growth. Some also incorporate deactivation kinetics to predict long-term reactor performance. While progress has been made, there is still no consensus on a universally applicable kinetic model, reflecting the complexity of the process.
Economic Considerations: The Path to Commercialization
Despite its promise, methane catalytic cracking is not yet economically competitive with steam methane reforming. The key to improving its economics lies in two areas: increasing hydrogen yield and finding valuable uses for the produced carbon.
High methane conversion rates are crucial for process efficiency. This requires highly active and stable catalysts, as well as optimized reactor designs. On the carbon front, producing high-quality carbon nanotubes or other valuable forms of carbon could significantly improve the process economics.
Some economic analyses suggest that if carbon capture and storage become mandatory for steam methane reforming, methane cracking could become the more economical option. However, this depends on factors such as the price of natural gas, the value of carbon products, and potential carbon taxes.
Looking to the Future
As we strive for a low-carbon future, methane catalytic cracking stands out as a promising technology for clean hydrogen production. While challenges remain, particularly in catalyst stability and reactor design, ongoing research is steadily addressing these issues.
The potential of this technology extends beyond just hydrogen production. The carbon byproduct, if properly harnessed, could find applications in fields ranging from electronics to construction, creating additional value streams.
As we move forward, interdisciplinary collaboration will be key. Advances in materials science could yield better catalysts, while improvements in reactor engineering could enhance process efficiency. With continued research and development, methane catalytic cracking may well play a crucial role in our future energy landscape, providing a pathway to clean hydrogen without the carbon footprint.
Source: https://www.sciencedirect.com/science/article/abs/pii/S0360319910022500